By Gavin Madakumbura1, Chad Thackeray2, Alex Hall3, Park Williams1, Jesse Norris2, and Ray Sukhdeo2
Key Takeaways:
Climate change may be linked to roughly a quarter of the extreme fuel moisture deficit when the fires began.
The fires would still have been extreme without climate change, but probably somewhat smaller and less intense.
Given the inevitability of continued climate change, wildfire mitigation should be oriented around (1) aggressive suppression of human ignitions when extreme fire weather is predicted, (2) home hardening strategies, and (3) urban development in low wildfire risk zones.
1UCLA Geography Department, 2UCLA AOS Department, 3UCLA IoES
The January 2025 wildfires in the Los Angeles region comprise the most destructive known wildfire episode in the region’s history. We have watched the unfolding wildfires with concern, dismay, and grief. The devastation has been at a scale beyond what we could have imagined, and we extend our deepest sympathies to those who have lost their homes and loved ones.
It will require extensive research to fully understand the relative importance of the various factors underlying the fires, and how the factors interacted, but there is broad consensus as to what those factors might be: there was a buildup of fuels—i.e. vegetation—from 2022–2024, followed by a very warm summer in 2024. Then the winter rains that normally arrive in November and December largely failed to materialize. On top of all that, we saw a nearly unprecedented Santa Ana wind event that was critical for the rapid spread of wildfires beginning on January 7, 2025.
Here we begin to quantify how unusual these factors are, in the context of the natural weather and climate variability. There have been many claims made about the role of climate change in the wildfires, which is understandable given the public’s interest in this question. However, fully answering this question requires deep analysis. Here we offer a starting point for this analysis, by identifying factors where human-induced climate change is very likely to play a role in the wildfires, factors where natural climate variability is at play, and others where more inquiry is needed to make definitive statements. We also note that there are no known natural ignition sources at this time of year in the region, so the fires were almost certainly started by human activity of some kind. In this sense, the human origin of the fires is indisputable. But we will leave to others to ascertain what led to the fires’ initiation, and focus on the climate and weather factors that allowed the fires to become so large and destructive.
Note that this work has not been peer reviewed (a months-long process), and additional lessons are likely to emerge as additional analyses are carried out over time.
Two Wet Years in a Row
We begin by noting that the 2022-2023 and 2023-2024 water years were both very wet in the Los Angeles region, which led to a buildup of vegetation in wildlands. For example, at the downtown Los Angeles weather station, rainfall totals for both years were nearly twice the long-term (1877-2024) mean. To assess how unusual this succession of two very wet years in a row is, we show in Figure 1 a time series of the 2-year running mean of precipitation averaged over coastal Southern California (blue curve). Since 1980, the 2-year average precipitation for the period ending in mid-2024 was the fifth wettest since 1980. This period included the once-in-a-lifetime Tropical Storm Hilary in August 2023, which inundated the region during the usually dry summer months. An annual mean measure of plant density and greenness (NDVI; green curve) shows the plant activity does generally track precipitation totals. For the 2-year period ending in mid-2024, vegetation greenness was very high, comparable to some of the greenest periods since 2000. Thus, the hillsides and mountains surrounding much of Los Angeles the region entered the 2024 fall fire season with substantially more grass and chaparral fuels than is common.
A mechanism has been proposed whereby climate change may promote higher precipitation totals in the wettest years. As the globe warms, the atmosphere on average contains more water vapor, so across much of the globe, the strongest storms (areas of water vapor convergence) are expected to become more intense. In California, the wintertime atmospheric river storms that generate most of the region’s precipitation are projected to transport more water vapor as climate warms (Huang et al. 2020), at a rate of about 4% per degree F of warming. Given a current warming of 2.7 degree F since the pre-industrial period, this would amount to roughly 10-11% more precipitation in each atmospheric river event. Assuming the vegetation converts the excess precipitation to carbon in a proportionate manner, the vegetation would contain ~10% more fuel than if climate change had not occurred. However, there is no observational evidence that wintertime storms in California, or the atmospheric moisture transports feeding the strongest of these storms, have intensified to date (Williams et al. 2024), making the degree to which climate change has promoted the high precipitation totals in 2023–2024 highly uncertain this time.
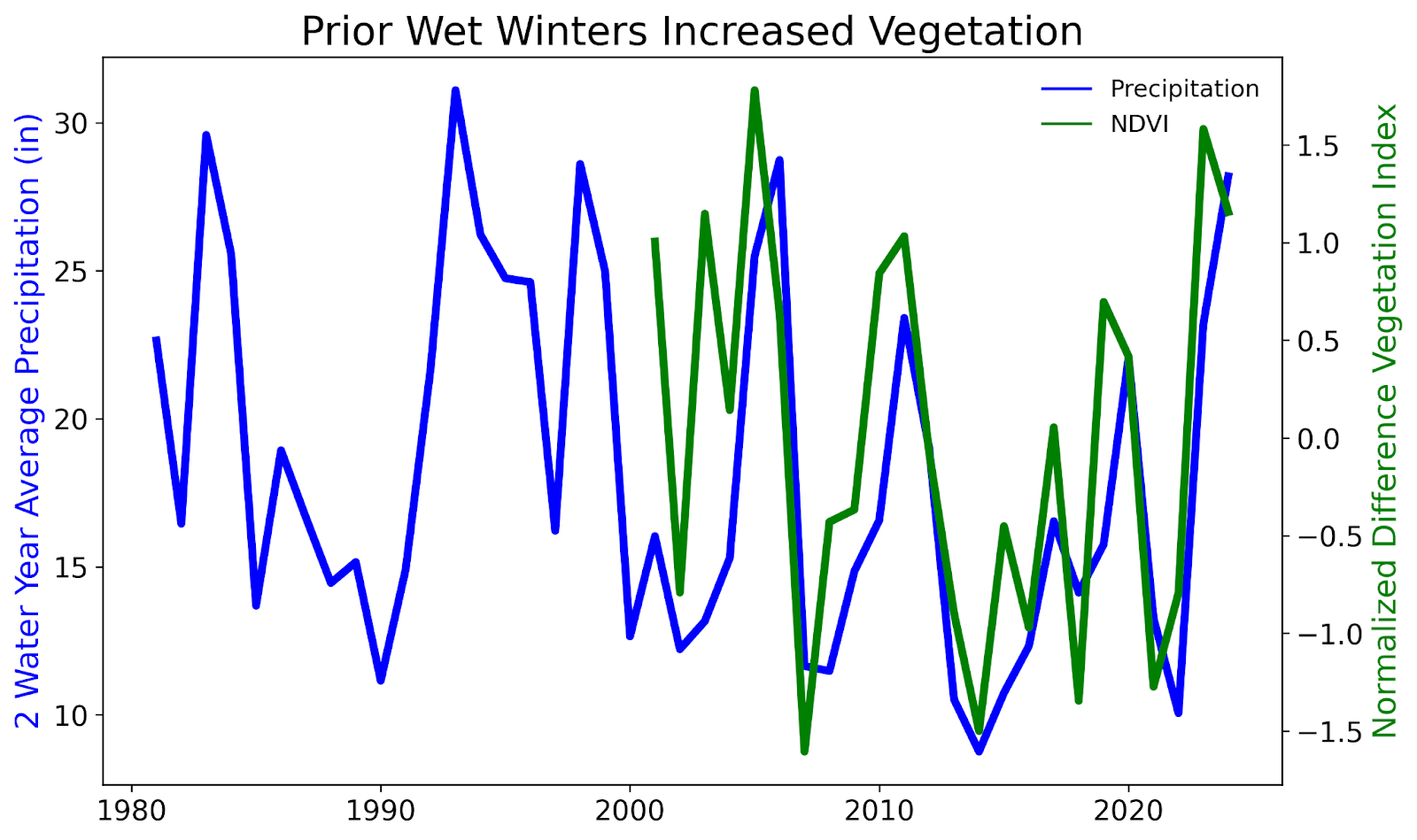
2024 Fuel Moisture Changes
The excess fuels available for burning in January 2025 are only the beginning of the story. Wildfires in Southern California typically feed on grasses and chaparral vegetation that dry out during the warm season. Outside of the high-elevation forests, the most dangerous and fast-spreading wildfires in Southern California are typically in the late summer and fall. This is because there is little-to-no rain through the summer while temperatures are high. By the end of summer, the fuels have lost most of their moisture. A measure of moisture levels in fuels is the “1000-hour dead fuel moisture”, or FM1000, which is an estimate of how much moisture remains in dead vegetation fuels such as tree stems and branches and is calculated from climate data. On average, the FM1000 in the coastal zone of southern California peaks in early March and reaches a minimum in August or September before the onset of the winter rainy season (Figure 2). In 2024, FM1000 was well above normal following the rains of winter 2023-2024 (on April 1st it was the 8th highest on record). However, it then exhibited a precipitous decline, reaching below normal levels by early summer, and further declining as the wet season failed to materialize. By January 7th of 2025, dead-fuel moisture was 6th lowest on record for that date. Thus, the antecedent conditions, both in terms of high fuel loads and low fuel moisture, were extremely favorable for wildfire.
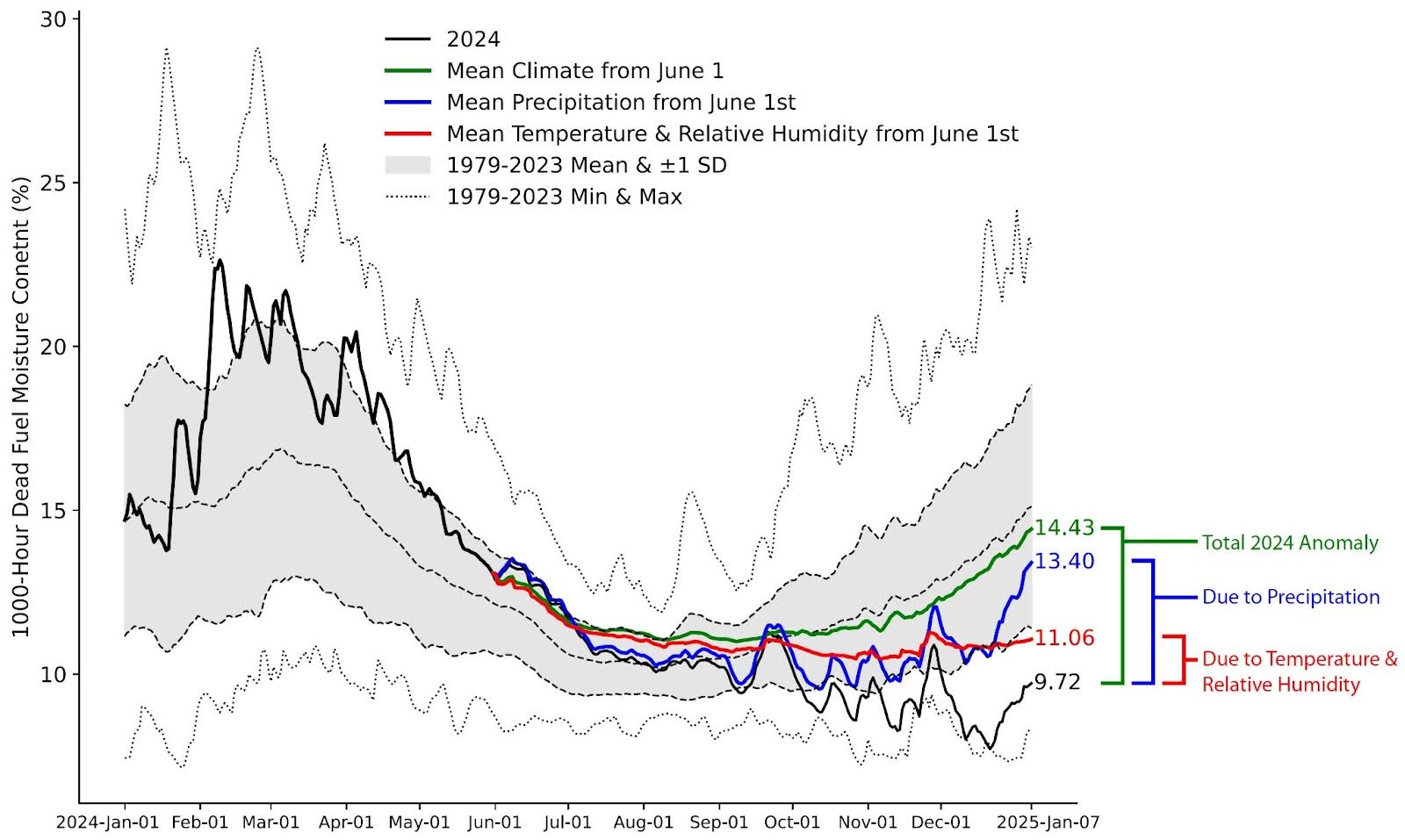
The Warm Summer and Fall of 2024
A likely factor underlying the extreme fuel aridity prior to the January fires was the anomalously hot summer-fall period of 2024. This may be particularly true for the moisture content of deeply rooted vegetation and human-made structures. While fine fuels such as grasses can dry out in a matter of minutes to hours in a Santa Ana wind event, larger fuels or vegetation with access to deeper soil-moisture reserves take longer to dry and therefore their moisture content can be the integration of climate anomalies over the course of months or longer. Heat can reduce the moisture content of fuels by increasing the rate at which the atmosphere can evaporate moisture from fuels and soil moisture. Following the wet winter of 2023-2024, the summer-fall of 2024 was exceptionally hot, including a record-breaking heatwave in September. In Figure 3 (bottom panel), we show that the summer-fall (June-December) of 2024, with a mean temperature of 67.1°F, ranks as the third hottest since at least 1895 in coastal Southern California. As we will demonstrate later, anomalous heat in summer 2024 appears partly responsible for the steep summertime decline in dead fuel moisture seen in Figure 2. It is noteworthy that other wildfires such as the Bridge, Line, and Airport fires followed on the heels of this unusual heat, underscoring the potential role for warming-driven aridification in these fires as well. The unusual warmth was part of a clear trend towards warmer temperatures over the entire 1895-2024 period (Figure 3, top panel). This warming is associated with global trends towards warmer temperatures, which have been attributed to human-induced climate change many times (e.g. the most recent IPCC assessment).
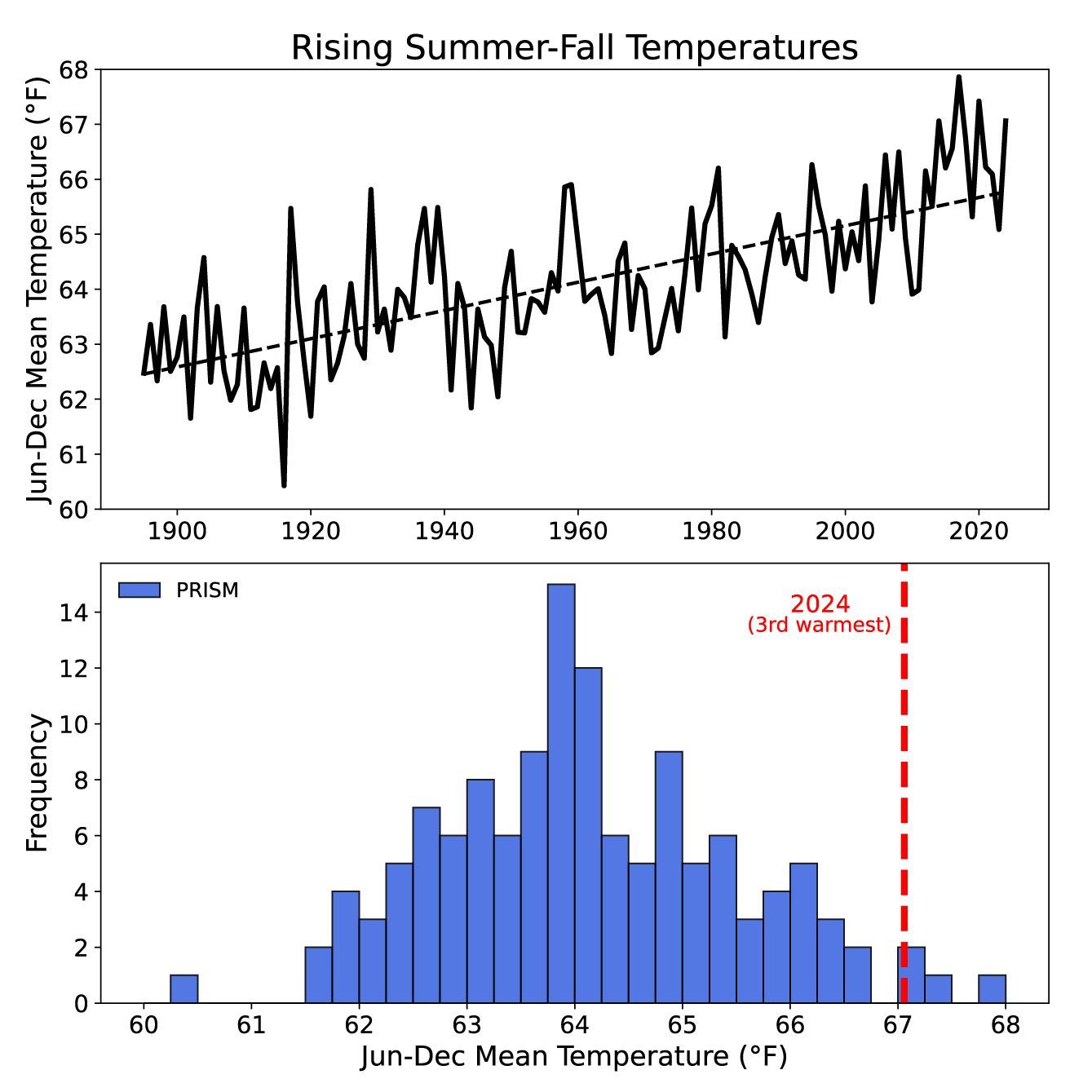
A Very Dry Start to the Wet Season
Another major factor in the aridity of fuels leading up to the January 2025 fires was the abnormal lack of precipitation. Typically the first significant precipitation event of the wet season arrives in the Los Angeles region between late October and early December, and yet at the time of the fires, the region had received no significant precipitation since April 2024. To examine this factor further, the top panel of Figure 4 shows precipitation totals accumulated by January 8 at the downtown Los Angeles station. This is the location for which we have the longest records in the region. The records here go back to 1877, allowing for a robust assessment of trends, as well the degree to which the current wet season is extreme. The 1877-2023 average accumulated precipitation total from May 1 through January 8 is 5.6”. However, precipitation totals during this period are highly variable. The one standard deviation range (~68% of the years) is 1.8”-9.4”, so that nearly a third of the years are either above 9.4” or below 1.8”. Even with extreme year-to-year variability being the status quo in this region, the precipitation total from May 1, 2024 through January 8, 2025 was extraordinarily low, only 0.29”. The extremely dry start to the 2024-2025 wet season is put further into context by the full distribution of all early wet season precipitation totals back to 1877 (Figure 4, bottom panel). The precipitation total of 0.29” as of January 8, 2015 ranks as second driest, behind only 0.15” in 1962-1963. It is also worth noting how rare it is for a dry start to the wet season to follow back-to-back wet years as we have just experienced. We find only three instances where an anomalously dry start to the wet season (<2.5” from May 1 to January 8) follows back-to-back wet (>16”) water years: 1907-1908, 1992-1993, and 2024-2025. Moreover, 2024-2025 is the only case with May-January precipitation below 2”.
The degree to which climate change may have promoted unusually dry, and as of now nonexistent, start to the wet season of 2024-2025, is highly uncertain. Climate models generally project a later start to the wet season in southern California (Williams et al., 2019, Goss et al. 2020), and indeed October–November precipitation totals in coastal Southern California have declined over the past six decades. On the other hand, the observed decline may be simply a regression to the mean after an extended period of unusually wet falls in the mid-1900s. At the downtown Los Angeles station, some of the driest starts to the wet season occurred in the early 1900s. More work is needed to better understand the potential role of climate change in the unusually dry start to the 2024-2025 wet season. In the meantime, we caution that interpretation of the potential contribution of climate change to a given precipitation anomaly in Southern California should be done so with the understanding that this region has an extraordinarily high range of natural variability of precipitation (Dettinger et al. 2011).
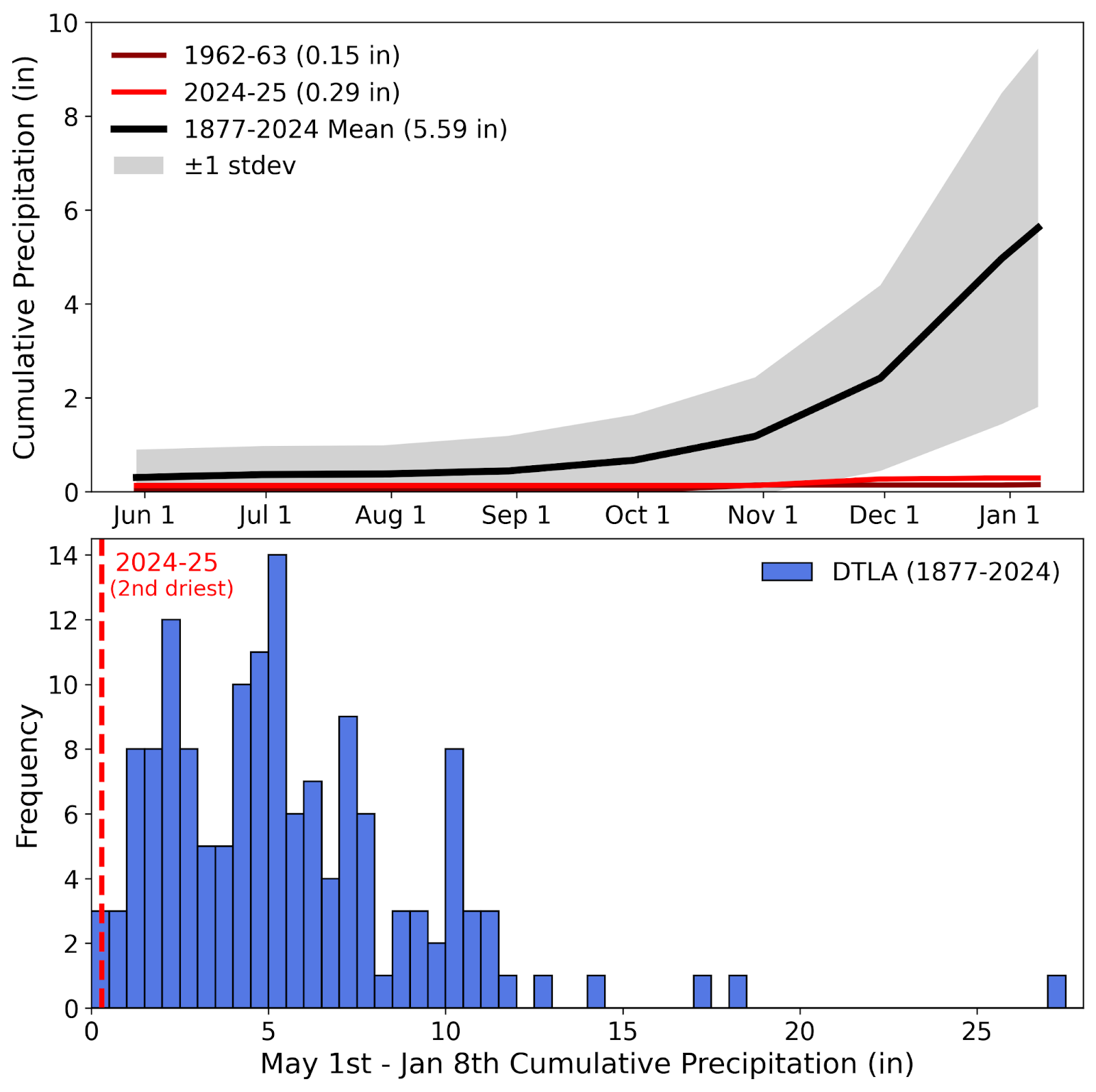
An Exceptional Santa Ana Event
Given the extreme aridity and fuel abundance in the wildlands around Los Angeles, the hurricane-force Santa Ana winds that arrived on January 7 were the final ingredient in a perfect recipe for major wildfire development. Santa Ana winds occur episodically in coastal Southern California from roughly October through March (Rolinski et al. 2019). Thus it is normal to have a succession of Santa Ana events in early January. The Santa Ana events’ high wind speeds, combined with the associated dryness of the winds, are known to increase wildfire risk in coastal Southern California (Keeley et al. 2024). Santa Anas are typically of greatest concern from a wildfire perspective in the first month or two of the October to March period, before the arrival of the first significant rains, typically in November (Cayan et al. 2022). However, because no significant rain had occurred when the January 7 event began, even an ordinary Santa Ana event occurring at this time would create fire risk comparable to an event occurring earlier in the season. In this sense, the region was still fully in “fall fire season” on January 7, despite the January label on the date.
So to what extent was the January 7-8 Santa Ana event out of the ordinary? This is a challenging question to answer, because winds are poorly observed compared to temperature and precipitation. High quality records that are representative of all of the fire-affected areas and extend back to the late 19th century are simply not available, and geographical coverage is relatively sparse, even for recent decades. Typically airport records have higher quality and go back further in time. So for the purposes of understanding how extreme the January 7-8 winds were, we rely on data from Santa Monica airport, Van Nuys airport, and LAX. Figure 5 shows the distribution of daily maximum hourly-averaged wind speeds for November through January Santa Ana days at each location. Although these stations are not in the heart of the Santa Ana wind corridors (Abel and Hall 2009), they still experience Santa Ana conditions during an event. The degree to which the wind characteristics are anomalous compared to the station’s climatology should be indicative of the regional event as a whole, especially if the stations agree. On January 7 and 8, maximum hourly-averaged wind speeds at Santa Monica Airport reached 25.3 and 21.9 mph (corresponding to the 98.9th and 96.7th percentiles of Santa Ana day wind speeds).
And at Van Nuys Airport maximum hourly-averaged wind speeds of 34.4 and 26.4 mph were recorded on the 7th and 8th, corresponding to the 99.4th and 91st percentiles, respectively. Note that because the above wind speeds represent hourly averages, the speeds associated with individual gusts would have been much faster. Similarly, very strong winds were observed further south at Los Angeles International Airport (98th and 87th percentiles on January 7 and 8, respectively). The collective picture that emerges from these three stations is that this was indeed a highly unusual Santa Ana event, though perhaps not entirely unprecedented. We did not find any statistically significant trends in wind speeds during Santa Ana days at these three locations. We are also hard pressed to name a mechanism whereby a warming climate would favor development of such an extreme Santa Ana event, and indeed the small body of research done so far suggests that climate change is generally associated with somewhat weaker Santa Ana winds in a warmer world (Abel et al. 2011, Guzman-Morales and Gershunov 2019). This may not preclude a role for climate change in intensifying the strongest events, but further evidence is needed to support a role for climate change in the extreme nature of the January 7-8 Santa Ana event.
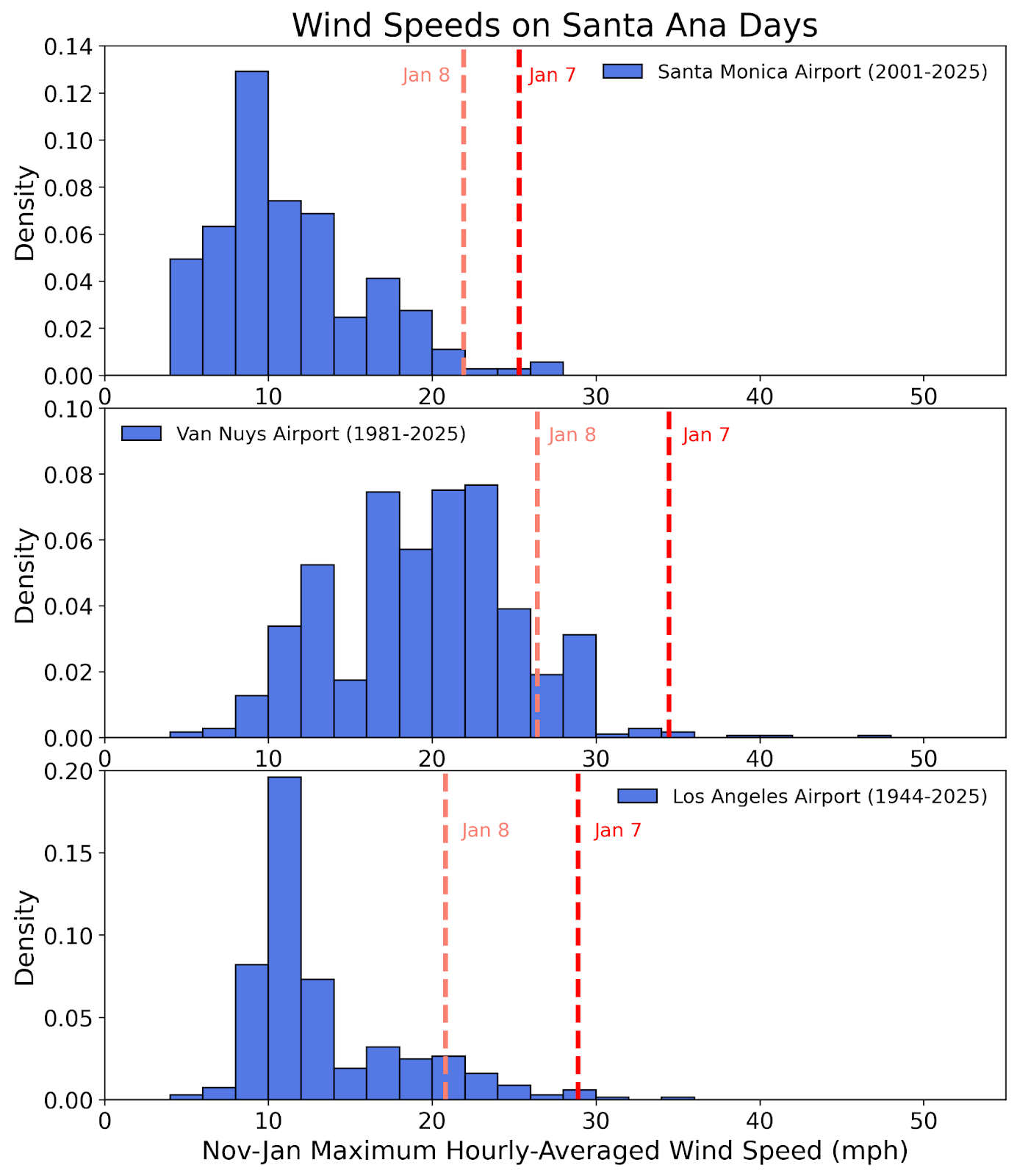
The Role of Climate Change
The clearest way in which climate change may have intensified the January 2025 wildfires is the anomalously warm summer and fall of 2024 (3rd hottest since 1895), and its drawdown effect on fuel moisture. However, the unusually low fuel moisture at the time of the fires is also strongly linked to the lack of early wet season precipitation, a factor that has likely arisen more from the large range of natural variability of Southern California precipitation than from human-caused climate change. Here we make an attempt to disentangle the effects of temperature versus precipitation anomalies by re-doing our calculation of fuel moisture (FM1000) for 2024 assuming alternative values for temperature, relative humidity, and precipitation from June 1, 2024 through January 7, 2025. Specifically, we first explored, with the green line in Figure 2, how FM1000 would have evolved if the wet winter and spring of 2024 had been followed by average climate conditions. We find that in this hypothetical case, the January 7 FM1000 value would be essentially equal to the long-term average. We then performed the same exercise twice more, once where June 1–January 7 precipitation follows its long-term average (blue line), and once where temperature and relative humidity (which is mostly a function of temperature) follow their long-term averages. The difference between the blue and black lines represents the drying effect of low precipitation totals since June 1. The difference between the red line and black line represents mostly the drying effect of the high summer and fall temperatures. The fact that both the blue and red lines are substantially different from the black (observational) line indicates that both low precipitation totals and anomalous heat were major contributors to the fuel-moisture deficit as of January 7. The offset between blue and black lines is approximately three times the offset between the red and black lines. This suggests the anomalous heat in summer-fall 2024 accounted for about 25% of the fuel-moisture deficit in early January 2025 and precipitation deficit accounted for the other 75%.
Note that the temperature anomalies assumed in the above analysis are relative to a baseline climate corresponding to the 1980-2023 time period. Substantial anthropogenic warming occurred prior to this period, so our estimate of the effect of heat anomalies in 2024 is conservative. We also noted above that a small part (~10%) of the excess precipitation from during the 2022-2023 and 2023-2024 wet seasons, which led to abnormally high fuel loads, may be climate-change-driven. This effect may be associated with climate “whiplash”, a phenomenon where climate change is simultaneously projected to intensify precipitation totals in wet years, but also to intensify drought in dry years (Swain et al. 2018, Swain et al. 2025). More research is still needed on this topic, and as noted above, the effect of past and future climate change on precipitation in California is highly uncertain (Williams et al. 2024). Finally, the extraordinarily strong Santa Ana winds on January 7-8, 2025 were crucial in driving the rapid rates of fire spread and there is little evidence that these extreme winds were promoted by climate change.
Further research is needed to understand how the factors above combined to produce the observed behavior of the January 2025 fires, including the overall contribution of the factors’ climate-change components. Based on current understanding of the importance of fuel moisture and fuel loads for wildfire behavior in grassland and chaparral ecosystems, we believe that the fires would still have been extreme without the climate change components noted above, but would have been somewhat smaller and less intense. Continued climate change is inevitable over the coming decades, and therefore so is the expectation of even more intense wildfires when all of the other necessary conditions for fire occur (e.g. fuel abundance, dryness, extreme winds, and ignitions). Thus wildfire mitigation should be oriented around factors we can control, and the damages we can prevent. These include (1) aggressive suppression of human ignitions in Southern California when extreme fire weather is predicted, (2) home hardening strategies to prevent structures from burning so easily, and generating embers that initiate fires in nearby structures, and (3) prioritizing urban development in zones of lower wildfire risk. Finally, we limited our analysis and discussion above to the January 2025 fires in southern California, but for a comprehensive assessment of the diversity of ways in which climate change and other factors have impacted wildfire in California to date, we refer the reader to Williams et al. (2019) and MacDonald et al. (2023).